Hello, readers. This is the concluding post on the topic I stopped in my previous post where I discussed about translocation and phloem.
Today, I will start by explaining the mechanism of translocation and its experimental evidence.
It is evident that the transport of material in the phloem is rapid,
usually 40-250 cm per hour, and can take place up or down the stem. How does
fluid now move in phloem vessels, in the absence of a heart of other organ to
create pressure? One widely accepted theory is the mass flow hypothesis or pressure
flow hypothesis, first proposed by E. Munch in 1930. This is represented below.
EXPERIMENTAL EVIDENCE FOR TRANSLOCATION
There are several different pieces of evidence to suggest that solutes made in the leaves are distributed to the rest of the plant via the phloem.
Ringing experiments
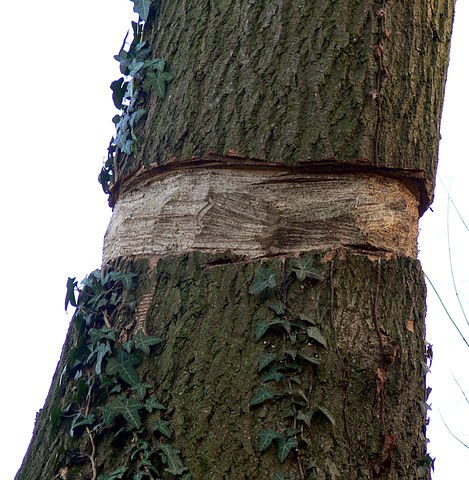
Phloem tissue of woody plants is situated on the underneath of the bark. If a ring of bark is removed, sugar will accumulate and cause a swelling above the ring, suggesting that sugar travels down the stem from the leaves to the roots. A similar thing happens if steam is applied in a ring around the stem. The living phloem cells are killed by the steam and so translocation stops, just as it does in the ringing experiment. The flow of water in the xylem vessels is unchanged, because the dead xylem vessels are unaffected by the steam.
Using radioactive tracers
If a particular leaf is given radioactive carbon dioxide (containing 14C), the isotope is incorporated into the sugars. After a while the stem is sectioned and X-rayed to show up the radioactivity, and the sugar shows up in exactly the pattern that corresponds to the phloem tissue. This technique will also show that sugars made in the leaves eventually accumulate in the growing points, the new leaves, and other sinks around the plant.
Using aphids
It is often difficult to get a pure sample of phloem sap to analyse, but aphids such as greenfly can locate and pierce phloem cells with great reliability and precision. If the aphid is then removed, leaving the stylet (needle-like mouth part) in place, pure phloem sap oozes from the cut end. Analysis of this fluid shows it to contain mainly sucrose with some amino acids and proteins.
LIVING IN EXTREMES
Plants need just four things in order to survive: water, light, a little warmth and a few minerals. If all four are available – even for a few days a year – plant life will exist. Plants have come to colonise many of the habitats of the world and, like animals, have become adapted for their particular environment. So far in this article, I have discussed plants that live in habitats where water is plentiful – such plants are known as mesophytes. However, there are many areas of the world where water is in short supply – drought – or where water is abundant but it is not available to the plant – this is physiological drought. Two examples of physiological draught are cold – where most of the water exists as ice – or in regions of high salt concentration, where the water potential makes it difficult for the plant to absorb the water. In this section we take a brief look at three plant types:
- Xerophytes: Plants that are adapted to arid regions.
- Halophytes: Plants that can tolerate high salt concentrations.
- Hydrophytes: Plants that live submerged or partially submerged in water.
XEROPHYTES – CONSERVING WATER IN DRY HABITATS
A xerophyte is a plant that is adapted to reduce water loss. The classic example is the cactus but many other plants show xerophytic adaptations. When water is in short supply, the transpiration rate seen in mesophytes cannot be supported. Xerophytes show a range of adaptations to minimise water loss while still being able to photosynthesise. These are summarised in the table below.
Adaptations of xerophytic plants
Adaptation | How it works |
swollen stem (e.g. cacti) | low surface area-to-volume ratio; acts as a reservoir for water |
leaves reduced to spines (cacti and Other succulent plants) | small surface area to reduce water loss; photosynthesis carried out by stem; spines protect stem |
rick cuticle (most xerophytes) | Waxy layer is impermeable to water – less evaporation |
low growth form | Some plants, even trees, can grow horizontally rather than vertically to avoid the worst of the wind |
leaf rolled inwards (e.g. marram grass) | stomata on inside – humid air trapped inside, where it cannot be blown away |
sunken stomata – in pits called crypts (e.g. marram grass) | as above, humid air is not blown away |
reduced number of stomata (most xerophytes) | reduced transpiration |
succulence – deep, fleshy leaves | leaves store water |
deep root systems | allow plant to reach water table that may be many metres below ground; many xerophytes have a high root-to-shoot ratio |
hairs (many cacti and marram grass) | reduce the air movement around the stomata, also protects from cold |
CAM photosynthesis | plants open stomata at night, absorb carbon dioxide and store it as an organic acid; then they close the stomata during the day and use the stored carbon for photosynthesis |
C4 photosynthesis | makes photosynthesis more efficient by reducing photorespiration; makes more efficient use of carbon dioxide and water |
short life cycles | plant can grow, flower and make seeds quickly when water is abundant – dormant seeds can survive the drought; these plants may not show other xerophytic adaptations |
Accumulate proline in cell vacuoles | this amino acid lowers the water potential so that more water can be absorbed from soil |
Leaves hang vertically (e.g.eucalyptus) | minimises evaporation and overheating by midday sun – photosynthesis mainly morning/later afternoon |
Cereus peruvianus (Cactus); A xerophyte. Augurar, CC BY-SA 3.0
HALOPHYTES
Globally, there is no substance that limits plant growth as much as salt as I have explained before. Halophytes (literally ‘salt plants’) have evolved in regions of high salt concentration. There are many different habitats that have problems with salt; ranging from cold, moist estuaries and salt marshes to hot, dry, salty deserts. Human activity has done a lot to increase the problems of salt – irrigation of dry areas can flush salt to the surface. Salty environments are areas of physiological drought- water is scarce just as it is in an arid environment.
Consequently, many halophytes share adaptations with xerophytes as is shown in the table below. The big problem with salt is that it lowers the water potential of the soil so much that water tends to be drawn out of a non-specialised plant. In order to survive, many halophyte species absorb salt (and therefore water), tolerating high concentrations or excreting the salt through the leaves.
Adaptations of halophytes
Adaptation | How it works |
cells accumulate salt | lower water potential in roots so that water can be absorbed from salty water |
salt glands in leaves | glands in the leaves secrete excess salt |
accumulate proline in cell vacuoles | this amino acid lowers the water potential so that water can still be absorbed (also seen in xerophytes) |
many xerophytic adaptations | see the previous table above |
HYDROPHYTES
Hydrophytes are flowering plants adapted for life in freshwater. There are very few flowering plants that can survive in the marine environment – seaweeds are not true plants. Life in water has the advantage of extra support and the obvious fact that water is not in short supply. However, gas exchange is more difficult – there is less oxygen for root respiration – and all but the clearest water can block out a significant amount of available light. Adaptations of hydrophytes are listed in the table below.
The flower of Nymphaea alba, a species of water lily. Wikimedia, Public Domain
Adaptations of hydrophytes
Adaptation | How it works |
Aerenchyma | air spaces in parenchyma tissue conduct oxygen down to submerged and anaerobic areas of plant; also helps flotation |
flexible stems and leaves | plant can bend with the flow of the water; water supports the plant – no need for strengthening tissue that would make the plant more ‘brittle’ |
breathing roots | aerial roots project up into the air to get oxygen |
reduced roots | no need for the roots to get water and mineral ions |
finely divided underwater leave | less resistance to the flow of water over the leaf |
FEED THE WORLD: ADAPTATIONS OF CEREAL CROPS
It may surprise you that over half the world’s food supply comes from grass. Not the green stuff that cows rely on, but grass seeds – rice, wheat and maize. Cereals are grasses that are cultivated for their seeds. There are about a quarter of a million plants species known to science, but these three provide us with more food than the rest of them put together.
The development of agriculture was a crucial step in the cultural evolution of humans. It marked the transition from a hunter-gatherer way of life to more permanent settlements, in which humans grew food plants and kept animals in paddocks. Cereals were among the first cultivated plants, and their selective breeding will be discussed in another post of mine.
SO, WHAT’S SO SPECIAL ABOUT CEREALS?
Cereal grains have a number of advantages over other plant foods:
- Cereals have a lower water content than most fruits and vegetables and are less water-demanding during growth. Thus they can be grown in more arid environments. Rice is a notable exception.
- The low water content means that cereals are easier to transport and store – they have a far longer shelf life than other plant products.
- They are very productive – yielding more food per area of land than other crops. Throughout the world, the most productive crop a farmer can grow is likely to be a cereal.
- They have a high protein content, 7-15 per cent.
In this section, I will look at each of the three staples; rice, wheat and maize.
A wheat field in Dorset, England. User:Steinsky, CC BY-SA 1.0
RICE
Most of the world’s rice is grown in waterlogged paddy fields. Rice-growing regions are hot with a heavy seasonal rainfall. The rice plant grows in heavy, silty soils that drain poorly. Consequently, rice has evolved to cope with being semi-submerged in an oxygen-poor environment.
Rice survives in waterlogged soils because its roots are able to respire anaerobically, and to tolerate the high ethanol levels that result. A specialised tissue, aerenchyma, allows oxygen to penetrate down into the roots. An advantage of waterlogged anaerobic soil is that cyanobacteria fix nitrogen, so less fertiliser is needed.
WHEAT
Wheat is a grass that has been cultivated since the earliest times of human settlement. It is grown all over the world in temperate regions. It needs quite a lot of water but is able to tolerate frost. Over the centuries, wheat has been selectively bred and has undergone dramatic genetic changes so that the modern varieties are unrecognisable from their ancestors.
MAIZE
Maize is a tropical crop that thrives in hot, arid conditions. It shows many xerophytic adaptations including C4 photosynthesis. Maize grows very rapidly. Leading to a harvestable crop just 3 months after planting seeds. C4 photosynthesis is much more water efficient than the C3 photosynthesis seen in most temperate crops – C4 plants produce the same amount of food for a smaller input of water.
CONCLUSION
After reading this chapter, and the previous ones on HOW PLANTS WORK: Tomatoes worth their salt, and HOW PLANTS WORK: Mycorrhizas, Xylem and Phloem, you should know and understand that:
- Roots have root hair cells to increase the surface area for absorption of water and mineral ions from the soil. Water is absorbed by osmosis, mineral ions largely by active transport. Many plants have a mutualism with a soil fungus, a mycorrhiza, that aids absorption. Water passes through to the centre of the root via two pathways. The symplast (‘living’) pathway consists of movement through cell cytoplasm, while the apoplast (‘non-living’) pathway consists of the spaces between cells and their walls. The endodermis surrounding the vascular bundle contains a casparian strip that blocks the apoplast pathway.
- Transpiration is the loss of water from the upper surfaces of a leaf. The transpiration stream refers to the passage of water though the plant, from roots to leaves, through the xylem tissue. Throughout the plant, from soil to atmosphere, water travels down a water potential gradient. Water potential is highest in the soil water and lowest in the atmosphere. Xylem tissues consists of dead, hollow tubular cells that connect to form a continuous conducting pathway. Water and mineral ions pass up the plant in the xylem.The cohesion-tension hypothesis explains how water passes up the xylem to the leaves. As water evaporates from the leaves it creates a tension in the column of water below. Water is cohesive (sticky), so a continuous column can be pulled up from the roots. Fluid in xylem vessels is therefore under negative pressure, so xylem vessels are reinforced by rings of lignin to prevent collapse.
- In most plants the stomata close at night to prevent water loss. The mechanism of opening can be summarised as: light → photosynthesis → ATP → potassium pumped into guard cells → water follows guard cells get more turgid, bend, pore opens. Most photosynthesis takes place in the leaves, which are the sources of organic molecules:‘food’. Areas of the plant that need ‘food’ are referred to as sinks.Examples of sinks include the growing points (meristems) at the tips of roots and shoots, flowers, fruits and roots.
- Phloem tissue is living (in contrast to xylem). It consists of two types of cell; sieve tubes that do most of the transporting, and companion cells that control the activities of the sieve tubes. The fluid in the phloem is rich in sucrose and amino acids. Movement of fluid in phloem occurs from source to sink and is called translocation. In contrast to xylem, this can happen up or down the plant.
- The mechanism of translocation is the mass flow hypothesis, or Munch’s theorem. Plants become adapted for different habitats. Mesophytes are plants that grow in areas of plentiful water. Xerophytes are adapted to arid environments. Halophytes can tolerate salt, while hydrophytes live in water.
REFERENCES
https://www.britannica.com/plant/xerophyte
http://ib.bioninja.com.au/higher-level/topic-9-plant-biology/untitled-6/water-conservation.html
https://en.wikipedia.org/wiki/Xerophyte
https://en.wikipedia.org/wiki/Halophyte
https://en.wikipedia.org/?title=Hydrophytes&redirect=no
https://study.com/academy/lesson/plant-adaptations-hydrophytes-mesophytes-xerophytes.html
https://en.wikipedia.org/wiki/Digitaria_exilis
https://en.wikipedia.org/wiki/Cereal
https://academicjournals.org/journal/JCO/article-full-text-pdf/CECB450571
https://en.wikipedia.org/wiki/Rice
https://en.wikipedia.org/wiki/Wheat
https://en.wikipedia.org/wiki/Maize
https://study.com/academy/lesson/plant-translocation-definition-mechanism.html
https://medical-dictionary.thefreedictionary.com/ringing+experiment
https://scienceaid.net/biology/plants/translocation.html
https://www.toppr.com/guides/biology/transport-in-plants/phloem-transport/
https://prezi.com/rmfpx9w6bxcs/evidence-for-translocation/
https://quizlet.com/77037265/biology-experimental-evidence-for-translocation-flash-cards/
If you appreciate the work we are doing, then consider supporting our witness @stem.witness. Additional witness support to the curie witness would be appreciated as well.
For additional information please join us on the SteemSTEM discord and to get to know the rest of the community!
Thanks for having used the stem.openhive.network app. This granted you a stronger support from SteemSTEM. Note that including @steemstem in the list of beneficiaries of this post could have yielded an even more important support.