greetings friends, always in life there is a transformation process where relativity is always present, well in this article I want to refer to the behavior of the second law of thermodynamics, although all mechanical work can be transformed into heat, not all heat can be transformed into mechanical work. so. What is the second law of thermodynamics? , I invite you to follow this very interesting publication where science predominates.
THE SECOND LAW OF THERMODYNAMICS
The inability of the first law to identify if a process can be carried out is remedied by introducing another general principle, the second law of thermodynamics. The first law does not restrict the direction of a process, but satisfying it does not ensure that the process will actually occur. When processes can not occur, this can be detected with the help of a property called entropy. A process does not happen unless it satisfies the first and second law of Thermodynamics.
The use of the second law of thermodynamics is not limited to identifying the direction of the processes. The second law also states that energy has quality, as well as quantity. The first law has to do with the quantity and transformation of energy from one form to another regardless of its quality. Preserving the quality of the energy is a main interest of the engineers, and the second law provides the necessary means to determine the quality, as well as the level of degradation of the energy during a process. Nature states that the total energy associated with a thermal source can never be fully and completely transformed into useful work. Hence, all work can be turned into heat but not all heat can become work.
SECOND LAW OF THERMODYNAMICS. CLASSICAL DEFINITIONS
Definition of Kelvin-Planck
"It is impossible to build an apparatus that operates cyclically, whose only effect is to absorb heat from a temperature source and convert it into an equivalent amount of work."
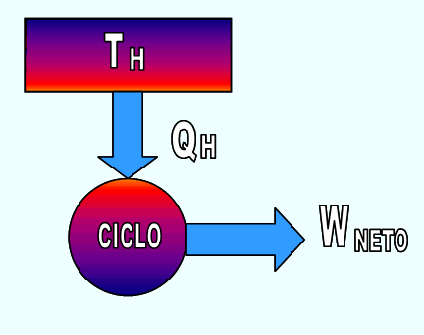
Definition of Clausius
"It is impossible to build an apparatus that operates in a cycle whose only effect is to transfer heat from a source of low temperature to one of higher temperature."
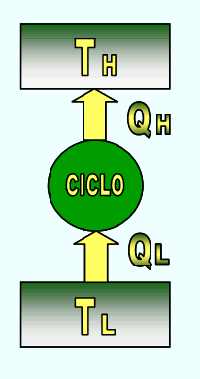
THERMAL MACHINE
It is a device that operates continuously or cyclically and executes a certain amount of work as a result of heat transfer from a high temperature source to a low temperature source.
The thermal machine allows to obtain a system that operates in a cycle with a positive work and a positive heat transmission.
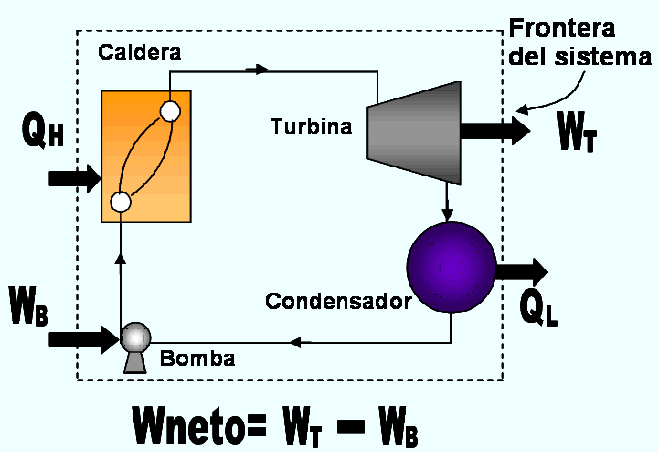
Figure 3. corresponds to a scheme of the steam power plant which fits into the definition of thermal machine. The scheme is quite simplified and the study of real steam power plants is studied at the point corresponding to the Rankine cycle. The different quantities shown in Figure 3. They are:
QH: amount of heat supplied to the steam in the boiler from a high temperature source (boiler burner)
QL: amount of heat released from the steam in the condenser in a low temperature sump (cooling water)
WT: amount of work delivered by the steam when it expands in the turbine.
WB: amount of work required to compress the water to the pressure of the boiler.
THERMAL EFFICIENCY
It is the measure of the performance of a thermal machine and is defined as the ratio between the net work obtained and the heat supplied to the working fluid.

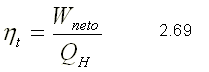
since the sum of the heats is equal to the sum of the works for a system that performs a cycle, the net work can be expressed as:

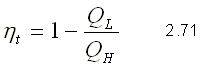
REFRIGERATOR MACHINE
It is a device that operates continuously or cyclically, requires work and carries out the objective of transferring heat from a body of low temperature to another of higher temperature. The working fluid used in the refrigeration cycle is called refrigerant. The most commonly used refrigeration cycle is the steam compression refrigeration cycle, which includes four main components: a compressor, a condenser, an expansion valve and an evaporator, as shown in Figure 4 . The refrigerating machine can work as a refrigerator or as a heat pump. Refrigerators and heat pumps operate in the same cycle, although they differ in objectives. The objective of the refrigerator is to keep the space cooled at low temperature by removing heat. The discharge of this heat to a medium of higher temperature is only a part of the operation, not the purpose. The goal of a heat pump is to maintain a warm space at high temperature. This is achieved by absorbing heat from a low temperature source, such as cold outside air, and supplying it to a high temperature environment such as a home.
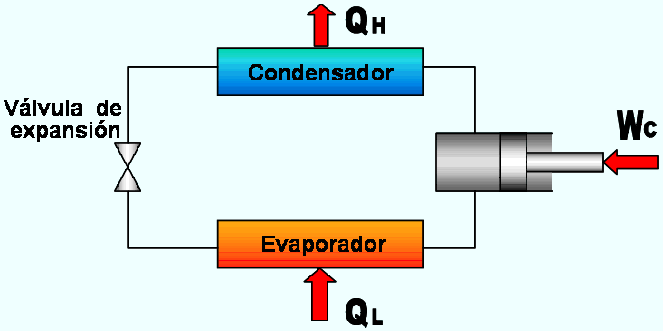
OPERATING COEFFICIENT
The efficiency of a refrigerating machine is measured with the operating coefficient that comes to be the equivalent of thermal efficiency in a thermal machine. For a refrigerator, the operating coefficient b is expressed by
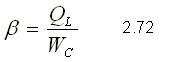
For a heat pump, the operating coefficient b is expressed by
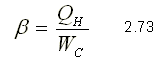
It is important to note that in a refrigerator the heat of interest is QL since this is the one that is extracted to cool a space. In a heat pump, the heat of interest is the QH since it is the one that is rejected to heat a space.
THE CARNOT CYCLE
It is a reversible cycle formed by four reversible processes which allow to obtain a greater efficiency of the cycle since the net work can be maximized by using processes that require the least amount of work and deliver the greatest amount of it.
Reversible cycles can not be achieved in practice because the irreversibilities associated with each process can not be eliminated. However, reversible cycles provide higher limits on the performance of real cycles. The thermal machines and the refrigerators that work in reversible cycles are models with which the thermal machines and the real refrigerators can be compared. Reversible cycles also serve as starting points in the development of real cycles and are modified as needed to meet certain requirements.
The Carnot cycle was proposed in 1824 by the French engineer Sadi Carnot. The cycle consists of four reversible processes, two isothermal and two adiabatic, and can be executed either in a closed system or in a stable flow, with pure substance or with a gas, Figure 5 . The four reversible processes that make up the Carnot cycle are the following:
Reversible isothermal expansion (process 1-2, constant TH with QH heat transfer to the gas).
Reversible adiabatic expansion (process 2-3, temperature decreases from TH to TL).
Reversible isothermal compression (process 3-4, constant TL with heat transfer from the gas).
Reversible adiabatic compression (process 4-1, temperature increases from TL to TH).
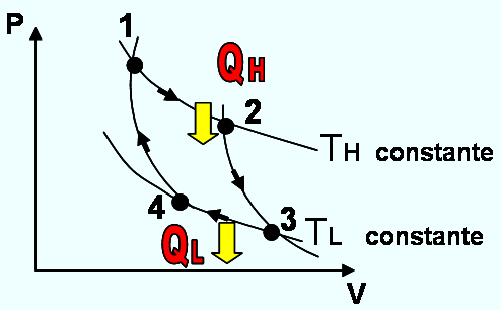
The Figure 5. corresponds to the Carnot cycle operating for a thermal machine, but all processes can be reversed to study the refrigerating machine. In this second case, the cycle remains exactly the same, except that the directions of any interaction of heat and work are reversed. Heat is absorbed in a quantity QL from the low temperature reservoir, and heat is discarded in the amount of QH in a high temperature reservoir, for which a work input is required.
A thermal machine that operates in a Carnot cycle is called a reversible machine. With this type of machine you get the maximum performance. No thermal machine that works between two given sources, can have a performance superior to that of a Carnot machine that works between the same sources. Figure 6.
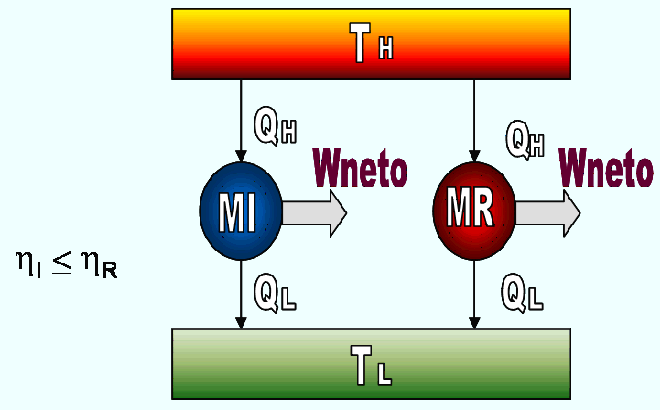
THE THERMODYNAMIC TEMPERATURE SCALE
As a consequence of the reasoning derived from the second principle, Kelvin proposed to use energy as a thermometric quantity to define temperature and justified the establishment and adoption of a temperature scale independent of the nature of the thermometric substance used. Based on the fact that the thermal performance of the Carnot cycle is independent of the properties of the fluid used and only depends on the temperatures of the sources, it could demonstrate that:
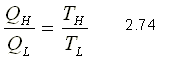
Therefore, the quotient QH / QL can be replaced by TH / TL for reversible devices, where TH and TL are the absolute temperatures of the high and low temperature reservoirs, respectively. Therefore for a reversible thermal machine, you can write
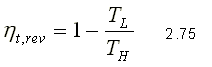
THE INEQUALITY OF CLAUSIUS
It was established for the first time by the German physicist R.J.E. Clausius (1822-1888) and is expressed as
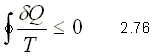
That is, the cyclic integral of d Q / T is always less than or equal to zero. The integration takes place over a complete cycle and can be reversible or irreversible.
If the cycle is reversible
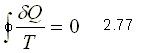
If the cycle is irreversible
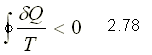
CONCEPT OF ENTROPY
The Clausius inequality is the basis for the definition of a new property called entropy.
It is considered a reversible cycle formed by two internally reversible processes A and B as shown in Figure 7.
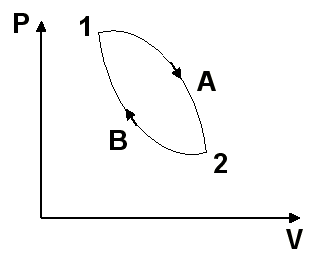
Applying the Clausius inequality, you have the following
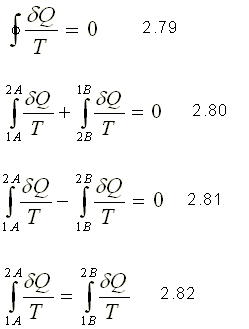
Since it is the same for both trajectories between states 1 and 2, the value of this integral depends only on the extreme states and not on the trajectory followed. Consequently, it must represent the change of a property since it is independent of the trajectory. This property is called entropy and is designated by S.
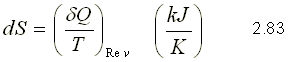
The entropy per unit mass, called s, is an intensive property and is measured with the unit kJ / (kg.K). The entropy change of a system during a process is determined by integrating equation 2.83 between the initial and final states:
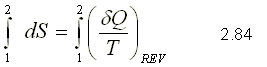
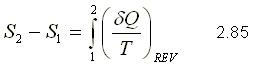
To perform the integration in equation 2.85, it is necessary to know the relationship between Q and T during a process.
CHANGE OF ENTROPY IN THE CARNOT CYCLE
In Figure 8. the Carnot cycle is represented in a T-s diagram. To do the entropy evaluation for each process, we start with the adiabatic compression process from 4 to 1; then with the isothermal heating process from 1 to 2; then with an adiabatic expansion of 2 to 3 and finally with an isothermal cooling process of 3 to 4.
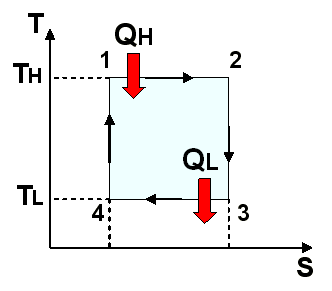
Reversible adiabatic process from 2 to 3
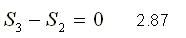
Reversible isothermal process from 3 to 4
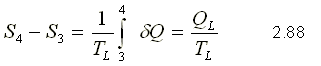
Reversible adiabatic process of 4 to 1

When the entropy remains constant during the process, that is to say S = 0, the process is called isentropic process.
CHANGE OF ENTROPY IN IRREVERSIBLE PROCESSES
In Figure 9. an irreversible cycle is considered formed by two processes, one reversible and another irreversible
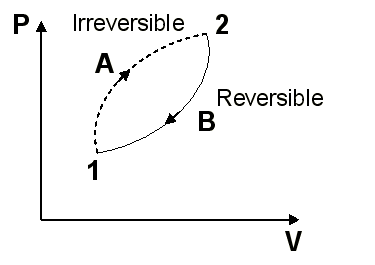
Applying the Clausius inequality
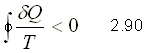
Transforming the cyclical integral into the sum of line integrals
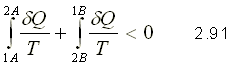
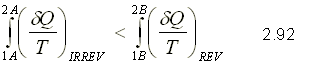
From equation 2.91 you can get to the following general expression
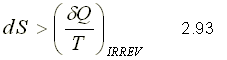
This expression corresponds to the entropy variation for an irreversible process.
PRINCIPLE OF ENTROPY INCREASE
The principle of entropy increase is expressed for any process like :

This principle is applicable both for closed systems and for control volumes. Equality is met for reversible processes and inequality for irreversible processes.
The entropy variation for the external environment is expressed as
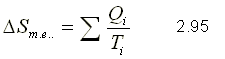
where Qi is the heat transfer across the boundary to the temperature Ti.
If the system is thermally insulated, that is, there is no heat transfer with the external medium, the principle of entropy increase is expressed as:
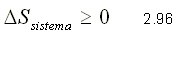
From this last expression follows the following conclusion: every isentropic process is adiabatic but not every adiabatic process is isentropic.
ENTROPY OF A PURE SUBSTANCE
Tds relationships are not limited to a particular substance in a particular phase. They are valid for all pure substances in any phase or combination of phases. For a pure substance, these relationships are too complicated, which makes it impossible to obtain simple relationships for entropy changes. Therefore, the values of s are determined from data of measurable properties that follow quite complex calculations and that are tabulated in exactly the same way as the properties v, u and h.
SECOND LAW FOR A CONTROL VOLUME
If, in the transport equation, entropy is replaced as an extensive property and then the resulting expression is introduced in the definition of entropy, the second law is obtained for a control volume.

Applications
Stable or steady flow
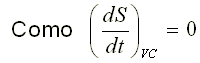

Transitory flow
Integrating equation 2.112, we obtain:

In general, according to the principle of increasing entropy:

The following video establishes a more explanatory concept graphically of the Thermodynamic Entropy:
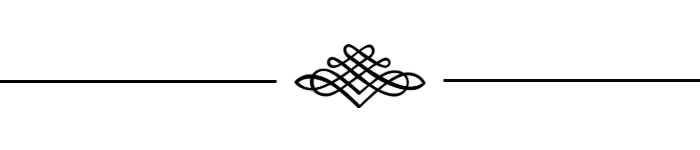
References
- H. Callen (1985) Thermodynamics and an Introduction to Thermostatistics, Wiley, NY.
- A. Bejan, (2006). 'Advanced Engineering Thermodynamics', Wiley.
- Denis J. Evans, E.G.D. Cohen & G.P. Morriss (1993). "Probability of second law violations in shearing steady states". Physical Review Letters 71 (15): 2401-2404.
- Denis J. Evans & Debra J. Searles (1994). "Equilibrium microstates which generate second law violating steady states". Physical Review E 50 (2): 1645-1648.
Bibliografia :
https://en.wikipedia.org/wiki/Second_law_of_thermodynamics
http://hyperphysics.phy-astr.gsu.edu/hbase/thermo/seclaw.html
https://www.theguardian.com/science/2013/dec/01/what-is-the-second-law-of-thermodynamics
https://www.grc.nasa.gov/www/k-12/airplane/thermo2.html
Adkins, C.J. (1968/1983). Equilibrium Thermodynamics, (1st edition 1968), third edition 1983, Cambridge University Press, Cambridge UK.
Atkins, P.W., Paula, J. (2006). Atkins' Physical Chemistry, eighth edition, W.H. Freeman, New York.
Attard, P. (2012). Non-equilibrium Thermodynamics and Statistical Mechanics: Foundations and Applications, Oxford University Press, Oxford UK.
@originalworks