Hello, my dear readers and members of this great community. Today, I will be discussing the electrode potentials under non-standard conditions, feasibility of reactions, cell potential, free energy, batteries and fuel cells.
ELECTRODE POTENTIALS UNDER NON-STANDARD CONDITIONS
So far, the assumption has been that the electrode potential reactions occur under standard conditions. That is, all solutions are 1.0 mol dm-3 in concentration, the temperature is 298 K and the pressure is 101 kPa. This is not the case for most reactions. Even if standard temperature and pressure could be maintained, as soon as the cell reaction starts, the concentrations of the reactants and products would necessarily change. The effect of changing conditions can be predicted qualitatively by assuming that the reaction taking place is an equilibrium and applying Le Chatelier’s principle.
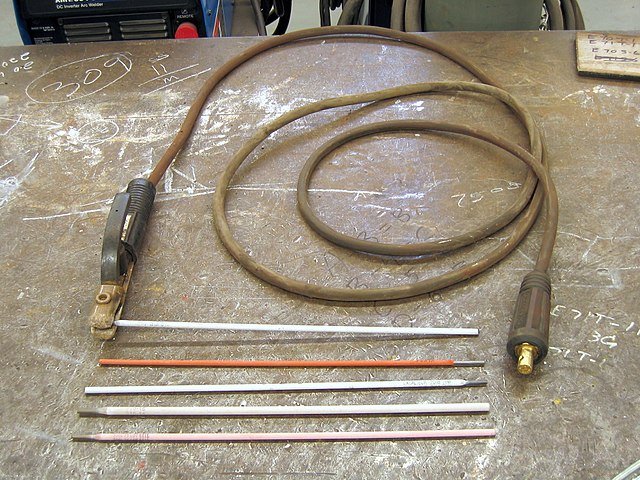
Temperature and cell potential
If the cell reaction is exothermic, then as the temperature increases the position of equilibrium shifts to the left. This means that the cell potential assumes a lower positive (or larger negative) value.
Concentration and cell potential
If the concentrations of the reactants are increased, then the position of equilibrium shifts to the right and the cell potential assumes a higher positive (or lower negative) value. If the concentration of one of the products increases, then the opposite effect is observed and the cell potential assumes a lower positive (or larger negative) value.
Concentration and electrode potential
Le Chatelier’s principle can also be applied to half-cells, to see how Eꝋred or Eꝋoxid values are affected. Consider the equilibrium between hydrogen ions and hydrogen:
2H+(aq) + 2e- ⇌ H2(g)
Under standard conditions Eꝋred = Eꝋoxid = 0.00 V
If the concentration of the aqueous hydrogen ion is increased, the position of the equilibrium shifts to the right and the reduction potential becomes positive. At the same time, the oxidation potential for the reaction becomes negative.
Pressure and cell potential
A change in pressure affects only those cell reactions that involve a gas. Essentially, increasing the pressure of a gas increases its concentration. Therefore, the effect of increasing the pressure of a gaseous reactant or a product on cell potential is the same as that with increasing the appropriate concentration.
Consider the hydrogen half-cell equilibrium again
2H+(aq) + 2e- ⇌ H2(g)
Under standard conditions Eꝋred = Eꝋoxid = 0.00 V
If the pressure of the hydrogen is increased, the position of the equilibrium shifts to the left. Therefore, the oxidation potential becomes positive and the reduction potential negative.
FEASIBILITY OF REACTIONS AND ELECTRODE POTENTIALS
The absolute value of the cell potential determines the feasibility of the reaction. When the cell potential is positive, the reaction is spontaneous. When it is negative, the reaction is not spontaneous. The description ‘spontaneous’ refers to the tendency of a reaction to occur. It says nothing about the rate of reaction. So it is possible for a spontaneous reaction to be so slow as not to occur. In an electrochemical cell, if Eꝋcell is positive, the reaction is spontaneous; if Eꝋcell is negative, the reaction is not spontaneous.
ELECTRON FLOW IN THE EXTERNAL CIRCUIT
As already stated, the external flow of electrons is from the anode to the cathode. The cell potential can be used to identify the anode. Provided the cell potential is positive, the cell works spontaneously and the anode is the electrode at which the oxidation occurs. One battery being developed uses liquid sulfur and sodium. The overall cell reaction is:
2Na + S → 2Na+ + S2-
The two half-equations are:
Na → Na+ + 2e- Eꝋoxid = +2.71 V
S + 2e- → S2- Eꝋred = +0.14 V
So, the cell potential is +2.85 V. This suggests that the cell reaction is proceeding in the correct direction. Therefore, electrons flow from the sodium electrode to the sulfur electrode.
Now, consider a cell that has a theoretical overall cell reaction of:
2Ag + Cu2+ → 2Ag+ + Cu
The half-equations are:
Ag → Ag+ + e- :Eꝋoxid = -0.80 V and Cu2+ + 2e- → Cu : Eꝋred = +0.34 V
So Eꝋcell = -0.46 V which indicates that, as written, the reaction will not proceed spontaneously. If the equation is reversed, then the cell potential becomes positive, which indicates that electrons will flow from the copper electrode to the silver electrode:
Cu(s) + 2Ag+(aq) → Cu2+(aq) + 2Ag(s) :Eꝋcell = +0.46 V
CELL POTENTIAL AND FREE ENERGY
Cells are a source of electrical energy that can be used to do work. A potential difference of 1 volt imparts 1 joule of energy to a charge of 1 coulomb. If the energy is used to do work, such as running an electric motor, then:
Work = charge × potential difference
In this case, the potential difference is the cell potential. Since the cell potential is necessarily positive in order to produce a current, the work done is negative (because charge is negative). This means that work is done on the surroundings.
Consider now the amount of work done when the molar quantities as shown in the overall cell reaction are used:
Work = (charge on number of electrons transferred during cell reaction) × Eꝋcell
The charge on the number of electrons transferred is the charge on n moles of electrons, where n is the number of moles transferred in the cell reaction.
Therefore:
Work = -nFEꝋcell
Where n is the number of electrons transferred in the cell reaction, and F is the Faraday constant, which is the charge on 1 mole of electrons in coulombs.
The work done according to this equation is the maximum amount of work that can be done. (In reality, some electrical energy is transferred by the heating of the wires.) The maximum amount of work available from a process carried out under standard conditions is known as the free-energy change, ΔG (G was chosen to celebrate the American Willard Gibbs, who developed the concept of free energy). So, in an electrochemical cell:
ΔG = -nEꝋcell
The unit of ΔG is J mol-1,
FREE ENERGY AND FEASIBILITY OF REACTIONS
Just as the cell potential must be positive for a reaction to occur spontaneously, so the value of ΔG must be negative. The free-energy change of reaction can be used in non-electrochemical cells to predict the feasibility of reactions. Any discussion of the feasibility of reactions must be taken with caution, since the Eꝋcell or ΔG values do not contain any information about the rate of reaction. Therefore, a reaction may have a large negative ΔG, but still not take place because its activation energy is too large.
BATTERIES AND FUEL CELLS
A battery is an electrochemical cell that is used as an energy source. Some kinds of battery have several electrochemical cells in series, and thereby combine the individual cell potentials to give a large potential at the output terminals. Batteries are normally designed to deliver a reasonably large current and to be able to withstand fairly rough handling. Batteries are of two types: primary cells and secondary cells.
PRIMARY CELLS
Primary cells cannot be re-charged. They are the most familiar type of battery on sale today.
LECLANCHÉ OR DRY CELL
The dry cell consists of a zinc anode (also the cell’s casing) and a graphite cathode. A paste of ammonium chloride, manganese (IV) oxide and zinc chloride surrounds the graphite electrode. The porous separator acts as a salt bridge.
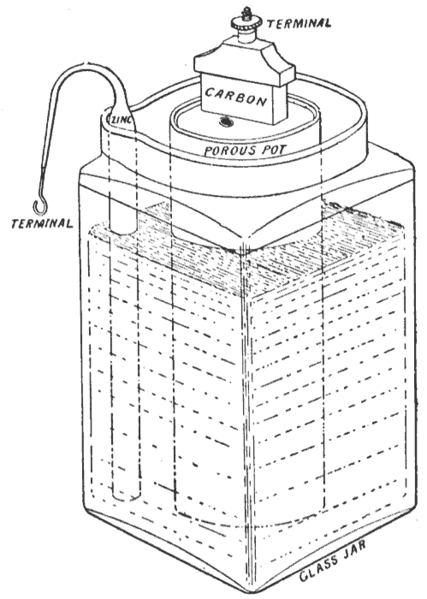
When the cell is connected into an external circuit, electrons flow from the zinc anode to the graphite cathode:
Oxidation half-equation: Zn → Zn2 +2e-
Reduction half-equation: MnO2 + NH4- + e- → MnO(OH) + NH3
The cell potential is about 1.5 V, which is impossible to verify using standard electrode potentials because the cell reaction occurs under non-standard conditions. The zinc ions present ions present in the paste prevent ammonia being given off by forming an ammine complex, [Zn(NH3)4]2+. In addition to the nuisance of having to replace this type of battery at frequent intervals, there is the disadvantage that the acidic ammonium chloride corrodes the zinc casing, even when the battery is not being used.
SECONDARY CELLS
These are batteries that can be recharged and therefore have a much longer life than primary cells. A typical rechargeable battery is the lead-acid battery used in most motor vehicles. The lead-acid battery consists of an aqueous electrolyte (30 per cent by volume sulfuric acid) and two sets of plates which form the electrodes. The positive plates are lead grids filled with a paste of spongy lead. The negative plates are lead grids filled with a paste of lead (IV) oxide. At first, the lead reacts with the dilute sulfuric acid to form an insoluble coating of lead (II) Sulfate, on both sets of plates. The battery is initially charged by direct current. During the charging process, the plates become chemically different. A layer of lead (IV) oxide is deposited on each negative plate, but the positive plates retain their layer of lead (II) sulphate.
The overall reaction during charging is: 2PbSO4(s) + 2H2O(l) → Pb(s) + PbO2(s) + 4H+(aq) + 2SO42- (aq).
Electrolysis takes place during charging. Once the battery is charged, the half-equations can be reversed to provide an external current or flow of electrons.
The overall discharging reaction is: Pb(s) + PbO2(s) + 4H+ (aq) + 2SO42-(aq) → 2PbSO4(s) +2H2O(l)
Note that sulfuric acid is used up during the discharging process and so its concentration decreases. This provides a convenient test for a lead-acid battery. Being proportional to concentration, the density of the sulfuric acid in the battery is simply measured with a hydrometer.
FUEL CELLS
A fuel cell is an electrochemical cell designed so that the reactants, often gases, are replenished continuously. This enables the cell to supply electric current continuously without recharging. A fuel cell is a much more efficient way of using a fuel to generate electrical energy. An electrical power station that burns a fuel is a very inefficient way of converting chemical energy into electrical energy. There have to be several energy transfer processes, all of which will reduce the efficiency of the overall energy transfer. With a fuel cell chemical energy is transferred directly into electrical energy.
The hydrogen-oxygen fuel cell is one of the most promising fuel cells being developed. The only chemical product from the fuel cell is non-polluting water; however, disposal of damaged or old fuel cells could cause heavy-metal pollution. One potential drawback at present with the hydrogen-oxygen fuel cell concerns the availability, transport and storage of liquid hydrogen. People think of the Hindenburg airship disaster in 1936 and they remain skeptical that the necessary safety measures can be put in place to ensure the safe use of hydrogen. No doubt in the future the use of hydrogen will become acceptable and fuel cells will be widely used.
HYDROGEN-OXYGEN FUEL CELL
A spacecraft needs a continuous supply of electrical energy. Much of it is provided by a hydrogen-oxygen fuel cell. Essentially, the overall cell reaction is that of oxygen and hydrogen to form water:
2H2(g) + O2(g) → 2H2O(l)
This is a redox reaction, in which electrons are transferred from hydrogen to oxygen. In a fuel cell, this transfer takes place via an external circuit, rather than directly during a collision between particles.
The electrolyte is aqueous sodium hydroxide. This is contained within the cell using porous electrodes, which allow the passage of water, hydrogen and oxygen. During their passage through the cathode, oxygen molecules are reduced to hydroxide ions, which enter the electrolyte.
Reduction half-equation: O2(g) + 2H2O(l) + 4e- → 4OH- (aq)
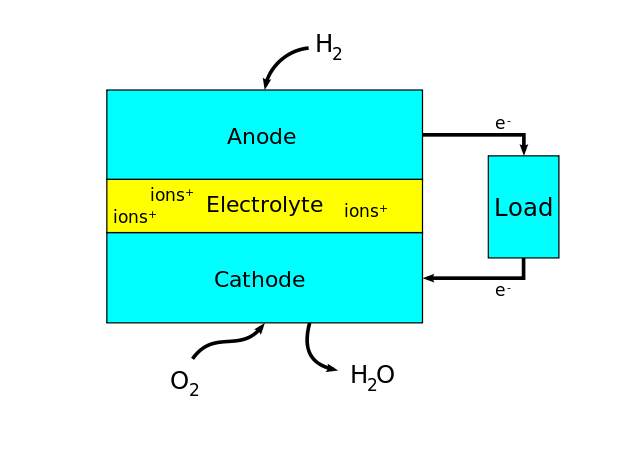
During their passage through the anode, hydrogen molecules are oxidised to give water molecules, and thereby hydroxide ions are removed from the electrolyte.
Oxidation half-equation: H2(g) + 2OH (aq) → 2H2O(l) + 2e-
Finally, fuel cells do not have to rely just on hydrogen and oxygen and research is proceeding to use methanol-oxygen fuel cells and even hydrocarbon-oxygen ones. Again the fuel cell comprises two half reactions producing a current without the need for combustion.
REFERENCES
- http://www.savitapall.com/Redox/Notes/Nernst%20Equation.pdf
- https://www.chem.purdue.edu/gchelp/howtosolveit/Electrochem/Electrochemical_Cell_Potentials.htm
- https://chem.libretexts.org/Bookshelves/Analytical_Chemistry/Supplemental_Modules_
- https://chem.libretexts.org/Bookshelves/General_Chemistry/Map%3A_Chemistry_.
- http://www.rod.beavon.org.uk/feasibility.htm#:~:text=Standard%20electrode%20potentials%20are%20widely,is%20regarded%20as%20being%20feasible.&text=The%20value%20of%20Eo%20is%20related,equilibrium%20constant%20for%20the%20reaction.
- https://www.quora.com/What-is-the-direction-of-electron-flow-in-an-electrolytic-cell
- https://socratic.org/questions/how-do-electrons-flow-in-a-galvanic-cell#:~:text=Electrons%20flow%20from%20the%20anode%20to%20the%20cathode%20through%20an%20external%20wire.&text=Here%20the%20Cu%C2%B2%E2%81%BA
- https://courses.lumenlearning.com/boundless-chemistry/chapter/cell-potentials/
- https://onlinelibrary.wiley.com/doi/pdf/10.1002/047173862X.app2
- http://www.docbrown.info/page07/delta3SGc.htm
- https://www.chemguide.co.uk/physical/entropy/deltag.html#:~:text=Whether%20or%20not%20a%20reaction,%CE%94G%20has%20to%20be%20negative.
- https://en.wikipedia.org/wiki/Leclanch%C3%A9_cell
- https://www.britannica.com/technology/Leclanche-cell#:~:text=zinc%2Dmanganese%20dioxide%20systems&text=zinc%2Dcarbon%20battery%2C%20also%20called,available%20low%2Dcost%20constituent%20materials.
- https://depts.washington.edu/matseed/batteries/MSE/classification.html
- https://en.wikipedia.org/wiki/Rechargeable_battery
- https://www.sciencedirect.com/topics/earth-and-planetary-sciences/hydrogen-oxygen-fuel-cells
- http://www.docbrown.info/page01/ExIndChem/electrochemistry11.htm
Thanks for your contribution to the STEMsocial community. Feel free to join us on discord to get to know the rest of us!
Please consider supporting our funding proposal, approving our witness (@stem.witness) or delegating to the @stemsocial account (for some ROI).
Please consider using the STEMsocial app app and including @stemsocial as a beneficiary to get a stronger support.