In the post of last week, I shared information about my research work on leptoquarks and associated theoretical calculations. This yielded quite a long post, which was technical here and there, and sometimes even hard to understand. This was made quite clear in the comment section of its French version (special thanks to @duke77 and @marc-allaria for their feedbacks). I will try to fix this issue with the current post. As always, (positive and negative) feedbacks are appreciated.
The subject of the day concerns again my own research. I will discuss a scientific publication from 2021 addressing toponium signatures at the Large Hadron Collider at CERN. This article has been published in an open access fashion (CC BY 4.0), so that you could download the original scientific publication if you want to.
Now it is time to move on with the topic and shed light on the word toponium…
In the first part of this blog, I will go back to the Standard Model of particle physics and the concept of quarks as building blocks of all atomic nuclei. As detailed last month, this idea results from a hundred-year-old story. Quarks and their antiparticles (called antiquarks) are known to form an authentic zoo of composite particles, as testified by decades of data in high-energy physics.
One exception is the top quark, the heaviest of all known particles. Top quarks decay well before having the time to form any composite state, so that the known particle zoo does not include any subatomic particle made of top quarks. Despite of this, quantum field theory magic leads to effects associated with virtual composite objects comprising top quarks.
Here, there is no formation of a real composite particle, as such a particle does not exist. In contrast, there are effects on measurable quantities that are similar to those that would originate from the presence of a composite particle made of top quarks. This is why we talk about a virtual object. These effects are however expected to be tiny, so that they should not be observable in data.
In the scientific publication to which this blog is dedicated, my collaborators and I demonstrated that the tiny effects coming from virtual particles made of top quarks could already be visible in published results from the Large Hadron Collider (the LHC). This contrasts with the expected paradigm, and could hence open the door to new discoveries. I will explain this point in the second part of this blog.
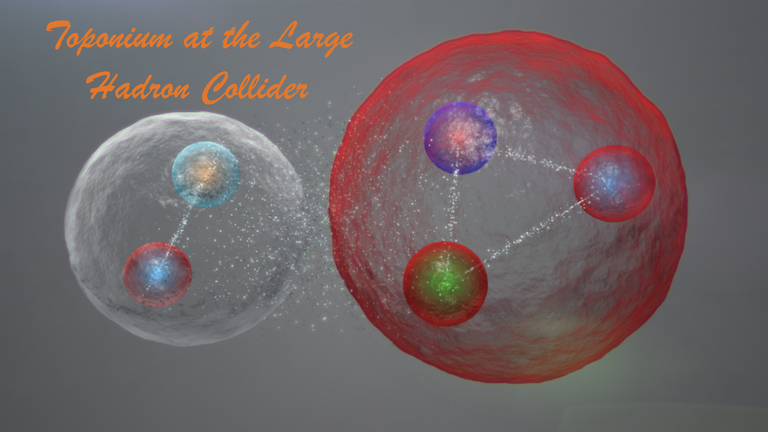
[Credits: CERN]
A window on the world of quarks
In this earlier blog, I introduced the Standard Model of particle physics and pointed out how it has been constructed during the last hundred years. At the end of the 1960s, physicists were left with a full zoo of subatomic particles (the more I use the word zoo the more I see it as appropriate). Pions, kaons, 𝛺’s, 𝜮’s, 𝜩’s, 𝜔’s, 𝚫’s were all over the place, which made the world of particle physics looking completely disorganised and messy.
In 1964 it was proposed that all those particles were not elementary, but instead composite objects made of several of eight elementary particles and antiparticles (the up, down, strange and charm quarks and antiquarks). This hypothesis turned out to be the good one (as verified by data), although nature showed us that we have in fact six elementary particles (the up, down, strange, charm, bottom and top quarks) coming each with an associated antiparticle.
This is what we can call ‘being organised’! With only a few elementary particles, we can describe all matter around us.
The question we may have at this stage is about how to link the elementary quarks and antiquarks to their bound states (a bound state of quarks and antiquarks is a composite particle made of quarks and antiquarks). The answer is driven by the theory of the strong interaction, called quantum chromodynamics.
In this framework, any quark has a given colour among red, blue and green. Correspondingly any antiquark has an anti-colour among anti-red, anti-blue and anti-green. Composite particles are then colourless (i.e. of a white colour). This shows that a colourless quark-antiquark pair can be formed from a quark of a given colour and an antiquark of the same anti-colour. In other words, a red quark plus an anti-red antiquark gives a white combination. Similarly, a blue quark plus an anti-blue antiquark and a green quark plus an anti-green antiquark both give a white combination too.
Quantum chromodynamics hence implies that particles made of a quark-antiquark pair can exist in nature. They actually do, and are named mesons. A meson comprises any of the five lighter quarks (up, down, strange, charm and bottom quark) and any of the five lighter antiquarks (up, down, strange, charm and bottom antiquark). A good list with most mesons is given on the associated Wikipedia page, which includes more than hundred entries! Somehow, mesons are everywhere, although all of them are unstable and do not live long.
In order to make a first link with the title of this blog, a meson containing a charm quark or antiquark is called a charmonium (the plural form is charmonia), whilst a meson containing a bottom quark or antiquark is called a bottomonium (the plural form is bottomonia). For what concerns toponium systems, we will discuss them in the next paragraph. They are related to the top quark, and none of the composite particles discussed so far includes any top quark. There is of course a good reason for this.
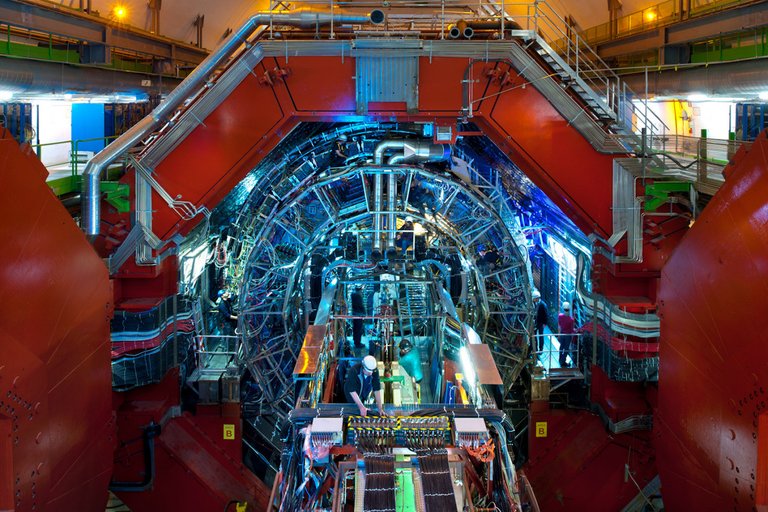
[Credits: CERN]
Whilst there are several other ways to construct white objects, this is not relevant for the present blog. I therefore only mention the example of combining three quarks of different colours. Red plus blue plus green indeed equals white. Such combinations are called baryons, the two most famous ones being the proton and the neutron. An almost complete list can be again obtained from the related Wikipedia page.
The specificities of the top quark
As already mentioned, we have six quarks and six antiquarks in the Standard Model. However, for everything said above the top quark and antiquark do not count. The theory indeed prevents us from having any composite particle containing a top quark or a top antiquark. The reason is very simple. The top quark is very heavy, with a mass reaching 175 times the mass of the proton. Due to such a large mass, the top quark can decay into a W boson and a bottom quark. This differs from all other quarks that are too light to decay into a W boson (and an additional quark).
The existence of this decay mode in a W boson makes the top quark very unstable, with a lifetime of about 10-25 second (this is equal to 0.0000000000000000000000001 second; yeah that’s fast!). This has profound implications. One of them is that once produced top quarks do not have time to form any composite state. In other words, the top quark is so unstable that it decays well before being able to pair with another quark or antiquark to form a meson or a baryon. Toponium mesons hence do not exist.
But then, we may argue that I had no reason to include the word toponium in the title of this post… Please don’t!
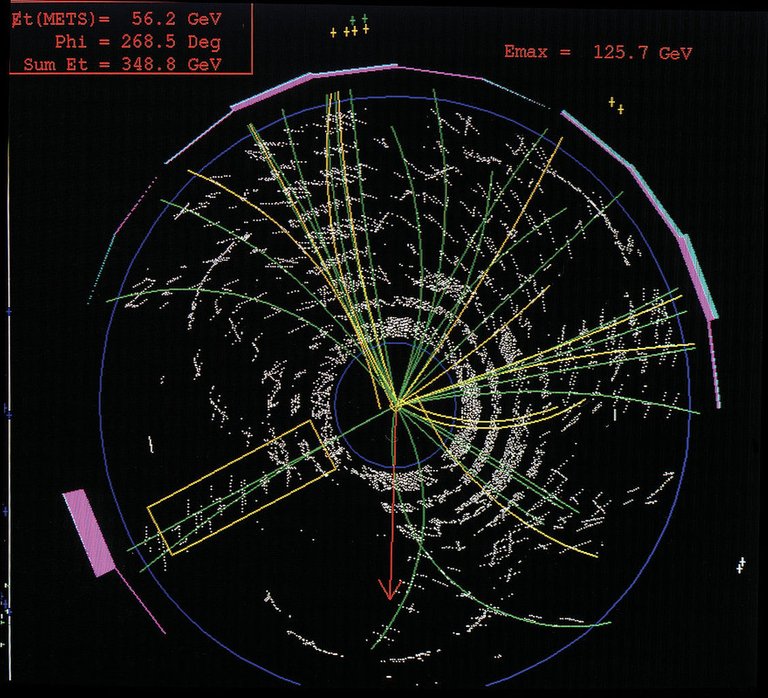
[Credits: Fermilab (public domain)]
The Standard Model of particle physics is of a quantum nature. I have already said that a lot in my last blogs. This means that we may have interesting quantum field theory effects in any phenomenon.
For instance, if we produce one top quark and one top antiquark at a particle collider such as the Large Hadron Collider at CERN, the two produced particles will quickly decay (in 10-25 second, as said above). However, it happens that they can strongly interact with each other before decaying. Relying on some calculations (for instance those, from 2010), we can estimate the associated effects. This is where it becomes interesting. When a top quark and a top antiquark are freshly produced and when they are both slow, then they behave as a composite system.
Composite systems made of a top quark and a top antiquark have however no time to form before the top and antitop decay. There is thus no real toponium particle appearing in the process. But if we focus on the effects associated with this configuration of a slowly-moving top-antitop pair, then it turns out that they are the same as those that would be expected from a top-antitop composite system.
In other words, toponium effects can potentially be visible although toponium does not exist. These earlier calculations from 2010 have estimated the importance of those effects on the rate associated with the production of a top-antitop pair at the Large Hadron Collider (the LHC). They have found that they lie at the permille level, which is much smaller than the experimental precision that could be expected on a production rate. More critically, knowing the rate at such a precision is probably not even achievable at all…
The conclusion from the above study from 2010 was thus somewhat funny: there is no need to worry about toponium effects, as those are invisible. Eleven years later, collaborators and I proved that this conclusion was too pessimistic.
Toponium at the Large Hadron Collider
The motivation behind my publication comes from recent top physics results of the ATLAS and CMS collaborations of the LHC.
As said above, the top quark is unstable, and decays almost instantaneously. Therefore, once produced at the LHC, a top-antitop pair immediately decays into other stuff that is observable in a detector. Physicists can then study the properties of those decay products. This is what is done in the pictures below (originating from results of the ATLAS collaboration).
I will not enter into details, and only focus on the part of the figure inside the red ellipse. Here, we can notice a slight disagreement between the data point (in black with its error bar) and the theory predictions (the sum of the coloured bars, with the theory error bar being represented as a hashed area). In the red ellipse, we can see that data exhibits an excess relative to predictions. The former is indeed higher than the latter on the plot.
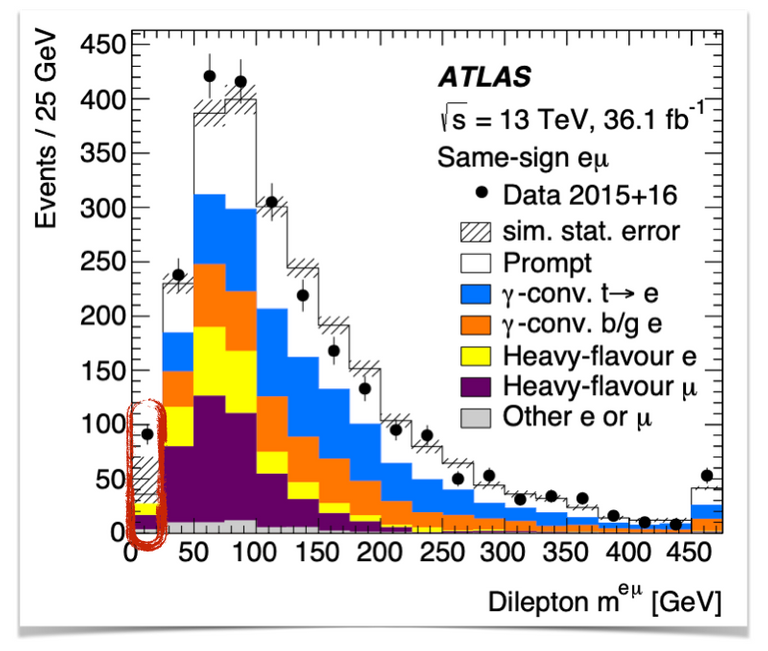
[Credits: EPJC 80 (2020) 528 (open access)]
This disagreement could be naturally thought of as a sign of a new phenomenon. But it could also be seen as something else: toponium effects. Whereas those effects are expected to be invisible on a total production rate, the above figure does not represent a total rate at all. First, there is a selection made on all collisions giving rise to top-antitop production. Once this event selection is made, then the above figure is extracted from data by focusing on a specific property of the top-antitop decay products.
What my collaborators and I found out and reported in our scientific publication from last year, is that a permille toponium effect can become a percent-level effect after event selection and once we focus on appropriate properties.
This consequently means that if we manage to control the experimental errors at the percent level, then it will become possible to observe toponium effects in data. Unfortunately, such a precision is still a bit far from what can be experimentally achieved.
We however didn’t get discouraged and started to tune the above-mentioned event selection. The idea was to find a manner to modify it in order to maximise the potential visibility of toponium effects. The nice part of our findings is that we managed to do so, as illustrated in the figure below.
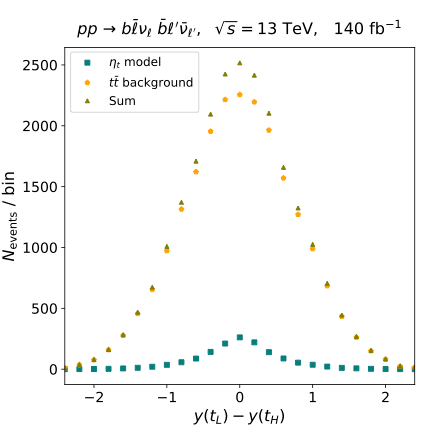
[Credits: PRD 104 (2021) 034023 (CC BY 4.0)]
This figure shows a property of the top-antitop decay products after a selection slightly different (but not too much different) from what is usually done in typical top-antitop production studies at the LHC. The orange circles show the chosen property when toponium effects are not included. The pure toponium effects are next represented by the teal squares, and the complete calculation (including toponium and non-toponium effects) is given by the olive triangles.
We see here that toponium formation contributes to hundreds of events (i.e.collisions) featuring the production and decay of a top-antitop pair. We have here a toponium effect of about 10%, which something impossible to miss! In other words, my collaborators and I demonstrated that there are handles to unravel the tiny effects associated with a virtual top-antitop composite particle. The trick is to focus on specific collisions featuring the production of a top-antitop pair, and consider given properties.
I didn’t read anything. What is all of this about?
In this blog, I discussed one of my recent research work, which ended up as an open-access publication in Physical Review D. The story funnily started at a conference in South Africa in 2020, during a breakfast (i.e. during the extra time outside the conference)… This was however in a pre-COVID world where it was still possible to travel…
We know that among all quarks of the Standard Model, the top quark is the only one that cannot form a composite system made of itself and other quarks and antiquarks. The reason is that it decays too quickly and have hence no time to pair with any other quark. However, sometimes, produced top quarks and antiquarks can behave as if they were part of a composite system. The associated effects are called effects of toponium formation, even if there is no real toponium around.
Calculations from a decade ago have shown that when we consider the production of a top-antitop pair at CERN’s Large Hadron Collider, those toponium effects are so small that they cannot be observed in data due to the experimental precision that will never be good enough. In my publication, we have shown that if we focus on the right property of the top-antitop decay products and that if we consider a specific subset of recorded collisions in which a top-antitop pair is produced, then toponium effects could have an impact of about 10%.
As this is something impossible to miss, our results have triggered the interest of the ATLAS and CMS collaborations of the LHC, and close discussions are on-going since then. The next experimental updates on the topic will hopefully confirm our predictions. However, we need to be patient before opening the bottle of champagne…
I hope you enjoyed reading this blog as much as I enjoyed writing it. Feel free to provide feedback of any kind (is it easy enough to follow, how can I improve my writings, etc.), to ask questions or clarifications, and to comment in general. Any form of engagement is always appreciated!
Cheers, and see you on Thursday for the French version of this blog and on Monday for a new story!
I found this essay easiest to follow since you started blogging again. That may be because this one builds on material already covered, or it may be because you explain the material better. I'm not sure. But I find it exciting that you and your colleagues have made a breakthrough that has roused interest in the theoretical physics community (even though it's not time to open the champagne yet).Hello @lemouth,
As I read about the 'invisible' (because of its rapid decay) top quark and that the only proof of its existence is data (the effect), I thought of an every day event. What if I watched someone who was about to swing a bat to break a glass, but I turned away for an instant, and didn't actually see the bat touch the glass. I'd hear the crash, see the fragments on the ground. Though I hadn't actually seen it happen, I would believe the bat hit the glass. What if I did that over and over again with the same outcome? I would have no direct proof that the bat broke the glass, but the circumstance and the effect would convince me.
You return to the issue of data, and its lack of precision. This seems to be the area in which you have made your breakthrough.
As you say, 10% cannot be overlooked.
I think the most impressive line in your whole essay for me is this:
That is an amazing statement, almost mystical.
A great lesson, @lemouth. Thank you. I hope I haven't mischaracterized anything you wrote. :)
Thanks a lot for the feedback. This is always appreciated! And what you wrote is fully correct!
I find it super exciting too. This topic has led in fact to my first article on Standard Model physics, all the rest of my research work lying in the context of physics beyond the Standard Model and new phenomena in particle physics.
We now pursue this work and try to provide better predictions ready to be used by our experimental colleagues. In this way, they will be able to directly compare our predictions with data (and conclude). Hopefully, this will allow for the addition of missing theory bits in different measurements for which there is a slight disagreement with theory, and which are expected to be sensitive to toponium effects.
What we try to do in the currently on-going follow-up study is to get more control on the theory predictions, and thus more precision (as mentioned later in your comment). For now, the 10% effects can be seen as an order of magnitude. They result somehow from a back-of-the-envelope calculation. What we now aim to do is to calculate this as precisely as possible, to know what is the true number and to be able to compare meaningfully with data.
It is actually an every day event. Pairs of top quark-antiquarks are produced with a very large rate at the Large Hadron Collider. During the previous run 2 (2015-2018), the LHC collected hence more than 100,000,000 top-antitop pairs. Although the top quarks decay instantaneously, their decay products leave tracks in a detector and can be reconstructed. From there, we can very certainly reconstruct the impact of intermediate top quarks and antiquarks.
The connection with the bat an the glass is a very good one.
Let's assume we take the Standard Model without the top quark. We can calculate how many times the glass gets broken, which produces glass fragments,. In other words, we can estimate how many times something could mimic the effect of a bat (i.e. of a top quark). Moreover, we can also analyse the configuration of the glass fragments, how they are relatively oriented, etc., and how many times we obtain a given configuration if we mentally reproduce the glass breaking experiment many many times.
But for now this was only the theory. Then come the measurements and the real experiment (let's break a few windows). We would find that there is no way to get the glass breaking observations and the rates with which each configuration of the fragment is occurring without having a bat (i.e. a top quark) in the process. Here is our proof (following the "sigma" convention we already discussed in an earlier blog).
To finish:
That is simply the Standard Model of particle physics, an extremely well-working framework but with room for decades of potential improvements. This is also the reason why I like this domain of physics so much :)
Of course it is...imprecise lay language. I really meant, more familiar to people who aren't scientists. More pedestrian. We gaze at the stars and wonder. You work with mysteries that may explain the universe.
I see! It's not just repeating the experiment, but calculating distinctions in outcome--data!
Thanks for a great, thoughtful response @lemouth.
You are very welcome!
lol. How creative.
Reading this, like agmoore says, was surprisingly easy to grasp. Parts of the way these particles behave seem reminiscent to how half-lives work in chemistry. But the amount of times i've read 'Quark' how now destroyed all meaning of the word for me... XD
Loosely related - assuming Time is functionally infinite, when working on these timescales, is there a supposition that, if you could access even smaller divisions of time, there would be more secrets to unravel that might undermine or advance your work?
Nice to see you here (and unexpected :) ). Thanks for your feedback.
Decay time and decay length are a bit different and here connected to a survival probability as a function of time. So yeah, there is a connection with what you remember :)
I can feel some irony here... But I guess this is well deserved. Physicists are not so good in names and acronyms, but sometimes a good one pops up.
I actually never asked myself why quarkonia (the generic name behind any quark-antiquark composite object) are called that way. I checked it out, and it turns out that this word comes from quark (obviously) and onium. The latter stands for a bound state of a particle and its antiparticle, according to Wikipedia. This is not very enlightening I must admit, but this comes from IUPAC who did that well before bound states became bread and butter....
I am not sure to understand this question, and I am afraid you will have to elaborate a little. Sorry :)
I'm trying to get back into being more active beyond my own postings these days =D I noticed the quality of content seems to be thriving... seemingly better than latter Steem days.
Well, since you work on such tiny timescales you presumably have extremely sensitive equipment to measure such things, combined with a lot of maths. But how short a timescale can particle physics currently, and usefully, work with? Close to Planck time?
Or, am I getting it wrong, and these timescales are only derived from maths?
Yes this is true, although the Steem bar was pretty low (I will always remember the banana story, one of the greatest moments of the good old Steem chat :D )
Those tiny time scales are in fact too small to be observed. From the electronic point of view, everything happens as instantaneous. What we actually see is the decay products of the top quarks and antiquarks. However, measuring the properties of these decay products allows us to conclude very solidly that we had intermediate top quarks and antiquarks in the process.
For that reason, we are very far from the Planck scale and not even close to it. The time scales there are predictions from quantum field theory (which be seen sometimes as math ;) ).
I could follow it easily. Almost as if somebody finally explained particle physics experiments in English. 🙂
I have few questions though. Mostly, regarding how I am trying to wrap my imagination around it.
Particle-antiparticle pairs are supposed to annihilate (like that owl, anti-owl did in discord). What stops a quark and an anti quark pair from immediate annihilation. Is the color force between them repulsive to hold them apart?
Is it known that decay time of a known particle can change when they form a composite particle. Say if t1 is decay time for particle x and t2 is of particle y. The decay time of xy is either t3 > t1 or t2 if it gets stable, or t4<t1 or t2 if it becomes more unstable? Is this what you think is happening with toponium, that it is getting more stable as a composite particle? Or you were able to see an even 10-25s small?
Thanks a lot for your feedback and the questions (which I will enjoy answering). You imagination is really cool here!
This works especially with owls (and @mathowl I guess)... More seriously, there are two items here.
Here there is no decay time of toponium because it does not exist. We see the effect of a bound state without having a real one. That's the (quantum field theory) magic behind it.
I hope this clarifies, although I am unsure I have fully answered the questions. Feel free to come back to me where needed.
Wow. Well thanks for the explanation. Let's see of I got this right. So itsince they are created in a pair formation, their initial state is moving away from each other. Also given that the strong force is weak at high energy, the quark and anti-quark don't come close enough.
Well a virtual particle showing it's effects! I mean I never though you can actually feel effects of something that didn't exist. Now what I am going to say may sound naive, but then my understanding of quantum physics is limited to very basics, so do forgive me. How does this magic exist? I mean something that never really existed, how can their effects pop into existence and become measurable? I know, I would need to read up on QFT probably to understand this. But is there a simple explanation that I can read/watch to wrap my head around this, which seems to be blown right now! 😁
Kudos to you and your team for the contribution made to the knowledge of particle physics. How is the toponium effect likely to affect our understanding of matter given the fact that with only a few elementary particle we can describe all the matter around us.
We can see the toponium story in four steps.
We can see that our understanding of how matter works is unchanged. It is more a matter that some effects were completely ignored so far, as assumed negligible. This is however not always the case.
Does it clarify?
Yes, your answer is clear and straightforward, but why did you choose the words potentially with respect to the toponium effects explaining the discrepancies between data and theory for various measurements related to top quark physics? Of course, I know that you wrote in your blog that it was not time to open the champagne bottle yet.
Toponium could explain what we have seen. This is what we have shown in our article.
To conclude about that fact, we need more precise theory predictions, as we only did back-of-the-envelope calculations. We are currently busy with those more precision computations, but they take time.
This is the reason why I used the word potentially (maybe there is something else hidden in data ;) ).
Thank you for the clarification sir.
:)
!discovery 30
Thank you! This is appreciated :)
This post was shared and voted inside the discord by the curators team of discovery-it
Join our community! hive-193212
Discovery-it is also a Witness, vote for us here
Delegate to us for passive income. Check our 80% fee-back Program
The rewards earned on this comment will go directly to the person sharing the post on Twitter as long as they are registered with @poshtoken. Sign up at https://hiveposh.com.
Thanks @agmoore to have shared this blog on Twitter, a social medium on which I have no account (people without any Twitter account still exist ;) ).
:)
I am on Hive so much that I have neglected Twitter. Every now and then I see something great (like this) that makes me remember there is another platform where I can get the word out.
I appreciate a lot that you have shared my blog! Really :)
Thanks for your contribution to the STEMsocial community. Feel free to join us on discord to get to know the rest of us!
Please consider delegating to the @stemsocial account (85% of the curation rewards are returned).
You may also include @stemsocial as a beneficiary of the rewards of this post to get a stronger support.
Congratulations @lemouth! You have completed the following achievement on the Hive blockchain and have been rewarded with new badge(s):
Your next target is to reach 69000 upvotes.
Your next target is to reach 12000 replies.
You can view your badges on your board and compare yourself to others in the Ranking
If you no longer want to receive notifications, reply to this comment with the word
STOP
Check out the last post from @hivebuzz: